Electrochemistry & Porphyrins
Porphyrins have emerged as exciting and powerful catalysts in electrocatalysis due to their versatile coordination chemistry and redox properties. This article provides an overview of recent advancements in porphyrin-based systems across key electrochemical transformations including oxygen evolution reaction (OER), oxygen reduction reactions (ORR), hydrogen evolution reaction (HER), and carbon dioxide reduction reaction (CO2RR). We explore their integration into frameworks, their role in redox flow batteries, and some innovative electrode functionalization strategies. Additionally, we provide insights into their structure-activity relationships, technological challenges, and potential applications.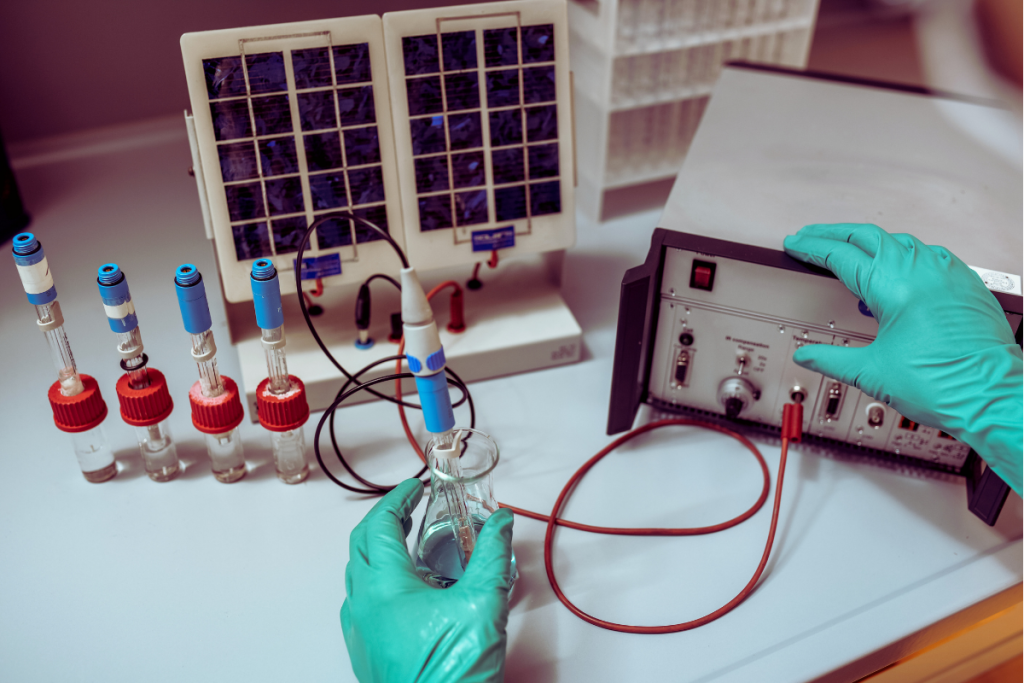
Introduction
Porphyrins are macrocyclic compounds which are essential components in certain biomacromolecules such as hemoglobin and cytochromes. These molecules coordinate metal ions, forming complexes capable of mediating electron transfer and small-molecule activation. This review focuses on porphyrin-based electrocatalysts, which play pivotal roles in sustainable energy applications, including water splitting, CO2 reduction, and energy storage. The ability to fine-tune electronic and structural characteristics by the modification of functional groups and metal centers makes porphyrins versatile candidates for tackling energy and environmental challenges.Did you know?
The foundation of electrochemistry was laid in 1800 when Alessandro Volta invented the Voltaic pile, the first true battery capable of producing a steady electric current. This milestone enabled scientists like Humphry Davy and Michael Faraday to discover and isolate chemical elements using electrolysis, leading to the formalization of electrochemical principles.
Porphyrin Structure & Activity in Electrochemical Reactions
The electrocatalytic activity of porphyrins stems from their conjugated macrocyclic structure, which coordinate many catalytically active metal ions and allow for precise tuning of electronic properties.
Factors influencing porphyrin performance include:
- Metal Centers: Transition metals such as Fe, Co, and Cu have demonstrated high activity in oxygen reduction reactions and CO2 reduction reactions due to their multiple redox states1
- Axial Ligands: The addition of axial ligands, such as imidazoles, can enhance electron density and stabilize catalytic intermediates, improving performance 1
- Substituents: Functional groups on the porphyrin periphery modulate electron distribution and hydrophobicity, impacting selectivity and stability
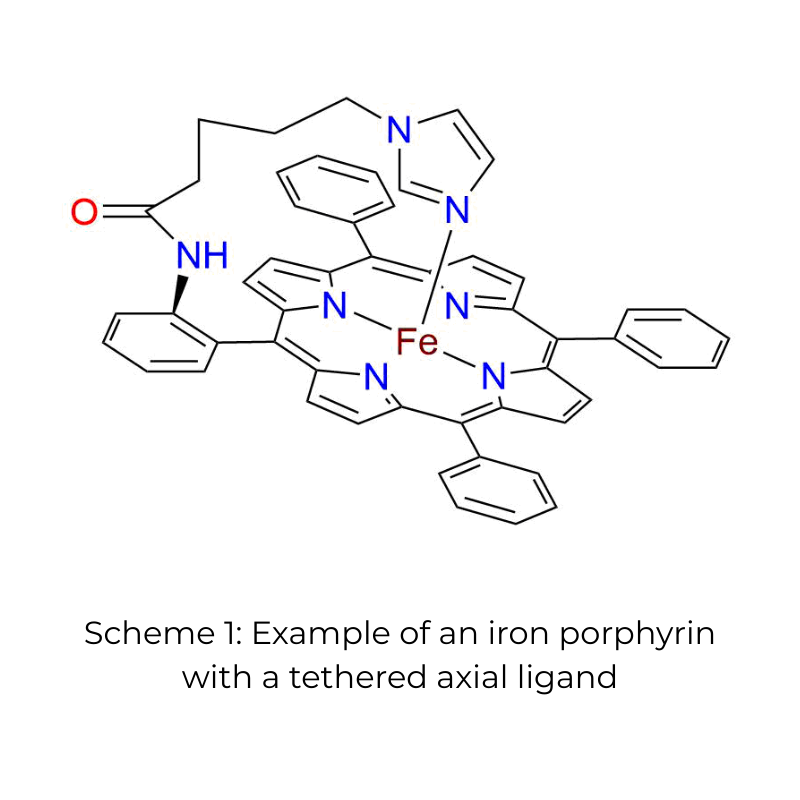
- Factors Determining the Rate and Selectivity of 4e–/4H+ Electrocatalytic Reduction of Dioxygen by Iron Porphyrin Complexes. Sudipta Chatterjee, Kushal Sengupta, Biswajit Mondal, Subal Dey, and Abhishek Dey. Accounts of Chemical Research 2017 50 (7), 1744-1753. DOI: 10.106/acs.accounts.7b00192
Porphyrin Applications in Key Electrochemical Reactions
Oxygen Evolution Reactions and Oxygen Reduction Reactions (OER and ORR)
Electrocatalytic water splitting is an electrochemical process that is being investigated for renewable energy conversion and consists of two simultaneous processes: oxygen evolution reaction (OER) and hydrogen evolution reaction (HER). The oxygen reduction reaction (ORR) and OER are essential for technologies like fuel cells and metal-air batteries. Precious metal based materials have been found to be the best materials for these processes but they’re costly and easily deactivate. Porphyrin based catalysts have achieved significant improvements for OER as this reaction typically suffers from high overpotential and slow kinetics. 2, 3, 4, 5
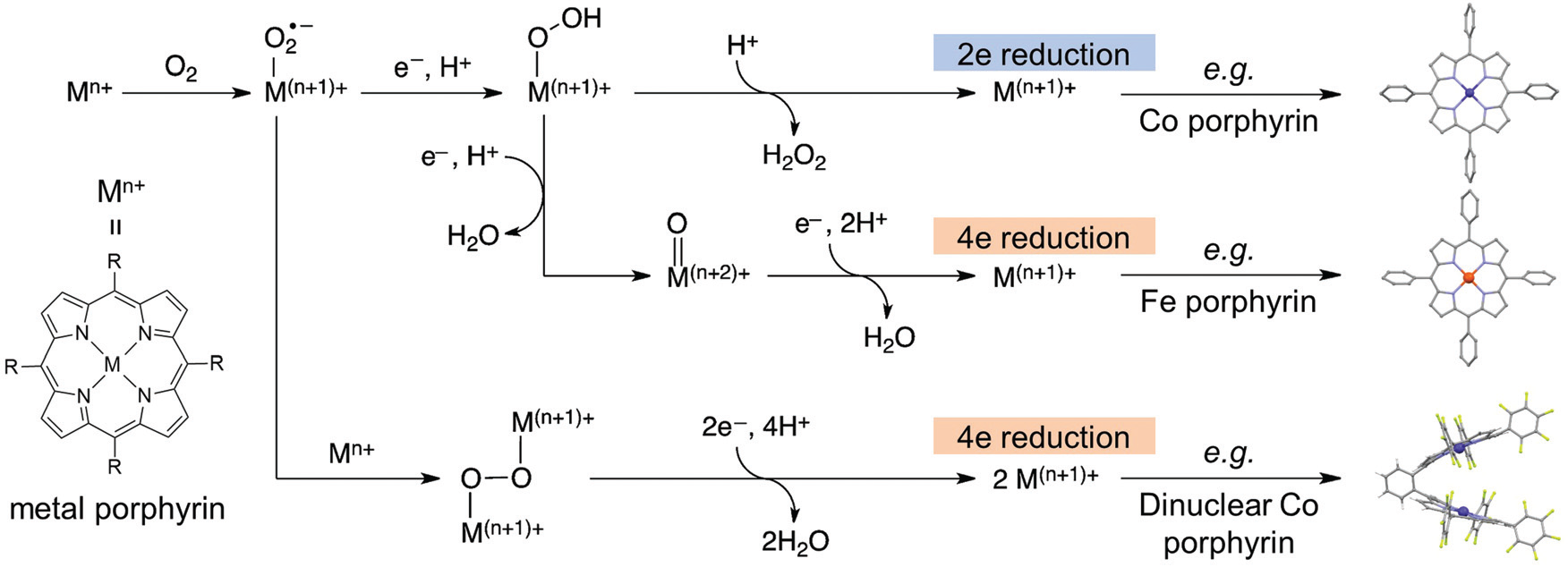
Scheme 2: Possible mechanistic
pathways for porphyrin catalyzed oxygen reduction reaction (ORR) taken from reference 4 (Liang et. al).
Enzyme-Inspired Designs:
Fe porphyrins with tethered imidazole ligands exhibit benchmark catalytic activity, rivaling Pt-based materials.
Scheme 3 (to the right) shows an example of an iron porphyrin with a tethered imidazole ligand.
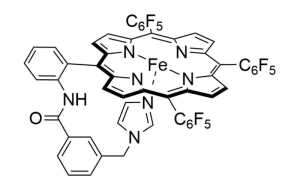
Framework Integration:
Embedding porphyrins in MOFs and COFs enhances their stability and electron transfer capabilities.
Below are some examples of porphyrins used in metal-organic frameworks (MOF) and covalent-organic frameworks (COF).
Compounds shown are meso-tetra(4-carboxyphenyl)porphine (T790), meso-tetra(4-aminophenyl)porphine (T40387), and meso-tetra(4-pyridyl)porphine (T618).
Hydrogen Evolution Reaction (HER)
First row transition metal porphyrin complexes have shown excellent activity for the hydrogen evolution reaction (HER) 6
- Mechanistic Insights: High turnover frequencies and efficiencies are attributed to optimized metal-ligand interactions. All metals screened gave Faradaic efficiencies of >97% for H2 generation during bulk electrolysis. The highest turn-over efficiency was observed for the Cu(II) complex with a turnover frequency of 48500 S-1.
- Challenges: Stability under acidic and alkaline conditions remains a limitation.
Need high quality porphyrins?
We offer hundreds of catalog and thousands of custom porphyrins in research and bulk quantities, ready to be shipped worldwide!
Here are some examples of first row transition metalloporphyrins used for hydrogen evolution reactions:
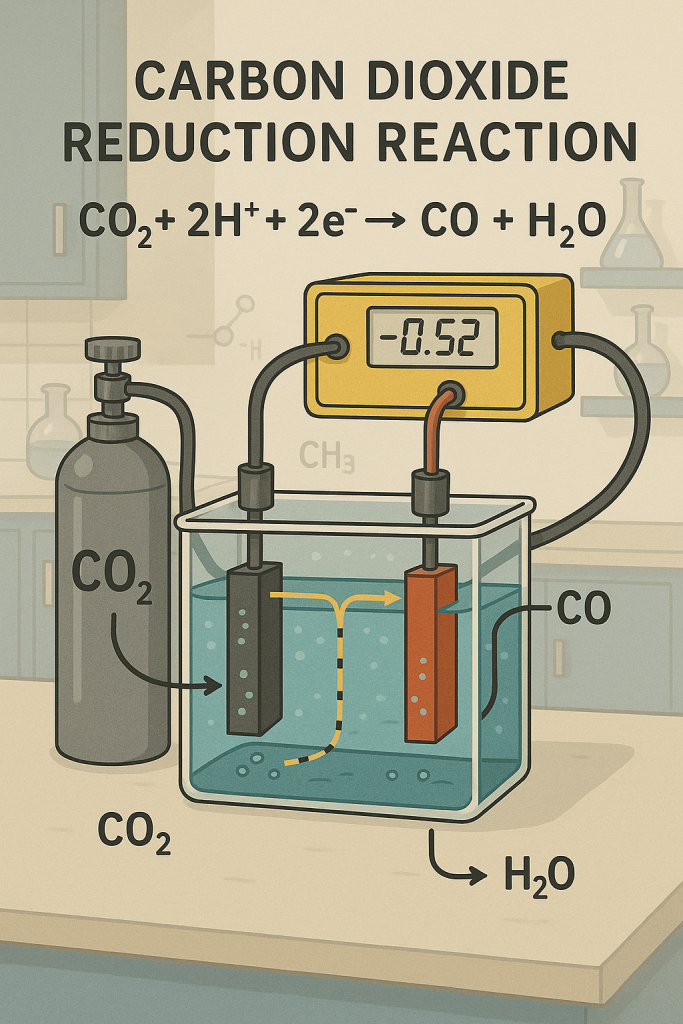
Electrochemical Reduction of Carbon Dioxide
- Catalyst Design: Functionalization of porphyrins with electron-withdrawing groups enhances CO2 affinity and reduction rates
- Scalability: Integration into electrodes addresses issues of solubility and reusability
- Formic Acid Pathway: CO2 + 2H⁺ + 2e⁻ → HCOOH
- CO Pathway: CO2 + 2H⁺ + 2e⁻ → CO
- Yao, B.; He, Y.; Wang, S.; Sun, H.; Liu, X. Recent Advances in Porphyrin-Based Systems for Electrochemical Oxygen Evolution Reaction. Int. J. Mol. Sci. 2022, 23, 6036. https://doi.org/10.3120/ijms23116036
- Graphite-Conjugation Enhances Porphyrin Electrocatalysis. Corey J. Kaminsky, Joshua Wright, and Yogesh Surendranath. ACS Catalysis 2019 9 (4), 3667-3671. DOI: 10.106/acscatal.9b00404
- Zuozhong Liang, Hong-Yan Wang, Haoquan Zheng, Wei Zhang and Rui Cao. Porphyrin-based frameworks for oxygen electrocatalysis and catalytic reduction of carbon dioxide
Chem. Soc. Rev., 206,50, 2540-2581. https://doi.org/10.1012/D0CS01482F - Enzyme-Inspired Iron Porphyrins for Improved Electrocatalytic Oxygen Reduction and Evolution Reactions. L. Xie, X.-P. Zhang, B. Zhao, P. Li, J. Qi, X. Guo, B. Wang, H. Lei, W. Zhang, U.-P. Apfel, R. Cao, Angew. Chem. Int. Ed. 2016, 60, 7576.
- Haitao Lei, Yabo Wang, Qingxin Zhang, Rui Cao, First-Row Transition Metal Porphyrins for Electrocatalytic Hydrogen Evolution, Journal of Porphyrins and Phthalocyanines, doi:10.1142/S1088424620500157
- Porphyrin Aggregation under Homogeneous Conditions Inhibits Electrocatalysis: A Case Study on CO2 Reduction. Kaitlin L. Branch, Erin R. Johnson, and Eva M. Nichols. ACS Central Science 2024 10 (6), 1251-1261.DOI: 10.106/acscentsci.4c0016
Porphyrin Frameworks in Electrochemistry
Porphyrin-based frameworks bridge homogeneous and heterogeneous catalysis.8, 9, 10, 11
The porosity, chemical, and catalytic properties of these materials can be modulated by changing the properties of the porphyrin building block.
Metal-organic frameworks and covalent-organic frameworks:
The synthesis of porphyrin-based metal–organic frameworks (MOFs) and covalent organic frameworks (COFs) typically involves the coordination of metalloporphyrins with suitable linkers to yield crystalline porous networks with high surface areas, that possess tunable electronic properties, and uniform active catalytic site distribution.
Hybrid Systems:
Examples of Porphyrins Used in Frameworks:
These porphyrins serve as modular and tunable building blocks in the design of advanced electrocatalytic materials, enabling precise control over the framework’s electronic environment and catalytic performance.
Iron(III) Porphyrin:
- Framework Type: MOF or COF
- Application: CO₂ reduction reaction (CO₂RR) and oxygen reduction reaction (ORR)
- Example: Fe–TCPP (iron(III) tetrakis(4-carboxyphenyl)porphyrin).
- Properties: The central Fe(III) ion acts as the catalytic site for redox reactions.
- Advantages: Exhibits high electrocatalytic activity due to the redox flexibility of the iron center and strong π-conjugation.
- Usage: Incorporated into MOFs like PCN-222(Fe) or COFs to create robust catalytic networks.
- Framework Type: COF
- Application: Electrocatalytic oxygen evolution and CO₂ reduction
- Example: Co–TAP (cobalt(II) tetra(aminophenyl)porphyrin)
- Properties: The cobalt center facilitates multi-electron transfer processes.
- Advantages: Shows good electrocatalytic activity and stability under harsh electrochemical conditions.
- Usage: Integrated into 2D COFs for high-density active site exposure and improved conductivity.
Scheme 6 (above) is taken from reference 9 (Gu et. al, 2022). We offer the following compounds:
- TCPP (first row) is T790: meso-tetra (4-carboxyphenyl) porphine
- tdcpp is T11766: meso-tetra (3,5-dicarboxyphenyl) porphine
- TCPP (third row) is T40779: meso-tetra (4-cyanophenyl) porphine
- TAPP is T40387: meso-tetra (4-aminophenyl) porphine
- TFPP is T34132: meso-Tetra (4-formylphenyl) porphine (custom porphyrin)
- TEPP is U34645: meso-Tetra (4-ethynylphenyl) porphine is available now if you contact us, but will be in our online catalog soon!
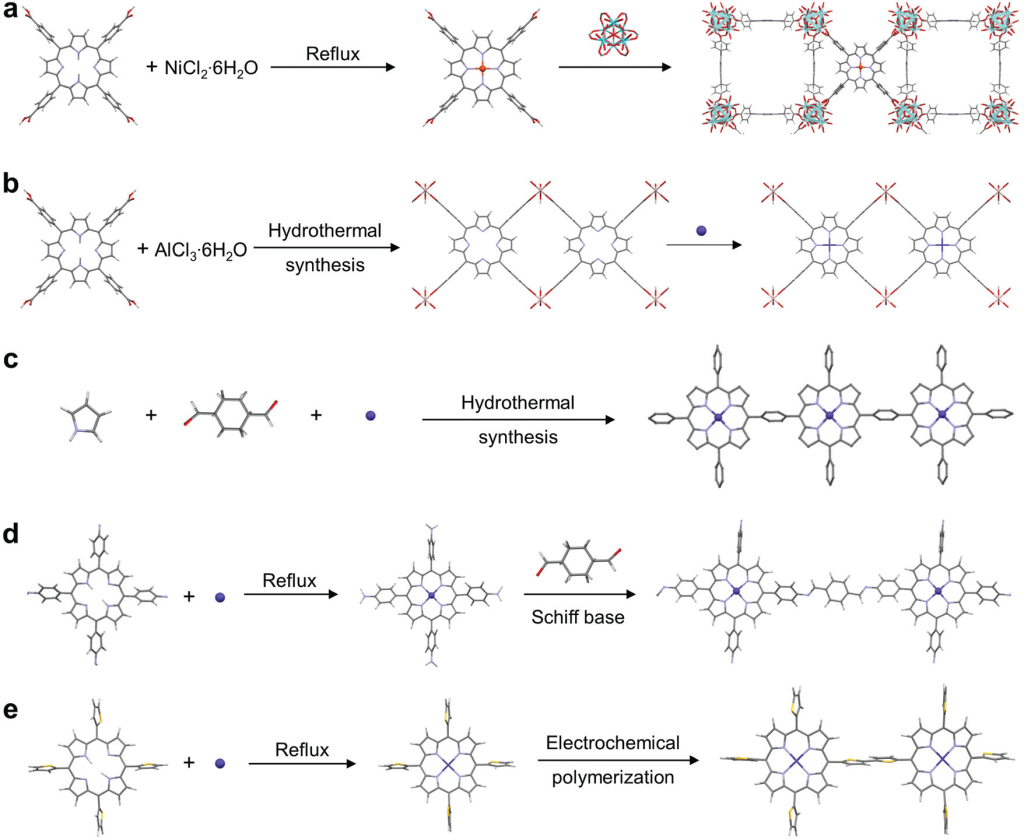
These frameworks are constructed using carefully selected molecular building blocks and crystal engineering techniques, enabling the control of framework topology and porosity, which is critical for optimizing electrocatalytic performance in reactions such as oxygen reduction (ORR) and CO2 reduction (CO2RR).
Synthetic strategies often focus on enhancing framework conductivity and accessibility by growing porphyrin frameworks on conductive substrates or incorporating them into hybrid materials to improve charge transport and mass transfer characteristics during catalytic processes.
Scheme 7: Synthesis of porphyrin-based metal-organic frameworks and covalent-organic frameworks, used from Zuozhong et. al (reference 8).
- Zuozhong Liang, Hong-Yan Wang, Haoquan Zheng, Wei Zhang and Rui Cao. Porphyrin-based frameworks for oxygen electrocatalysis and catalytic reduction of carbon dioxide
Chem. Soc. Rev., 206,50, 2540-2581. https://doi.org/10.1012/D0CS01482F - Gu J, Peng Y, Zhou T, et al. Porphyrin-based framework materials for energy conversion. Nano Research Energy, 2022, 1: 9120009. https://doi.org/10.26599/NRE.2022.9120009
- N. Lv, Q. Li, H. Zhu, S. Mu, X. Luo, X. Ren, X. Liu, S. Li, C. Cheng, T. Ma, Electrocatalytic Porphyrin/Phthalocyanine-Based Organic Frameworks: Building Blocks, Coordination Microenvironments, Structure-Performance Relationships. Adv. Sci. 2023, 10, 2206212. https://doi.org/10.1002/advs.202206212
- Catalytic Porphyrin Framework Compounds. Feng, Liang et al.Trends in Chemistry, Volume 2, Issue 6, 555 – 568. DOI: 10.1016/j.trechm.2020.01.003
Porphyrins in Electrochemical Energy Storage
Scheme 8 shows Electrochemistry of the TPP system. Used from Ma et. al (reference 12).
Redox Flow Batteries (RFBs):
Porphyrin-based RFBs operate efficiently under subzero temperatures unlike current commercial vanadium based aqueous redox flow batteries, showcasing high-capacity retention and stability.
This superior performance is attributed to the intrinsic molecular flexibility and reversible multi-electron redox capabilities of porphyrins, which enable efficient charge transfer even in harsh thermal environments. Additionally, porphyrin molecules can be synthetically tailored to optimize solubility, redox potential, and stability, allowing for greater design flexibility in electrolyte formulation. These advantages make porphyrin-based systems particularly attractive for renewable energy integration and grid-scale storage in cold climates.
- Challenges: side reactions with electrolytes and limited solubility need further investigation
Scheme 9 shows side reactivity of Fe(III) TPP with different electrolytes, taken from Mitchell and Elgrishi (reference 13).
- Porphyrin-Based Symmetric Redox-Flow Batteries towards Cold-Climate Energy Storage
T. Ma, Z. Pan, L. Miao, C. Chen, M. Han, Z. Shang, J. Chen, Angew. Chem. Int. Ed. 2018, 57, 3158. https://doi.org/10.1002/anie.201711523 - Investigation of Iron(III) Tetraphenylporphyrin as a Redox Flow Battery Anolyte: Unexpected Side Reactivity with the Electrolyte. Nathan H. Mitchell and Noémie Elgrishi. The Journal of Physical Chemistry C 2023 127 (23), 10938-10946. DOI: 10.106/acs.jpcc.3c01763
Challenges and Future Directions
While porphyrins have great promise, they also face challenges such as:
- Stability: Aggregation and degradation limit their long-term use
- Conductivity: Strategies to enhance electron transfer within frameworks are essential
- Scalability: Transitioning from laboratory-scale to industrial applications requires innovations in catalyst synthesis and integration.
Future efforts should focus on developing multifunctional frameworks, improving hybrid systems, and leveraging computational tools to optimize catalyst design.